Efecto de la luz, la humedad y la salinidad sobre el crecimiento de los organismos que componen la costra biológica del suelo
DOI:
https://doi.org/10.25260/EA.23.33.3.0.1968Palabras clave:
biocostras, cianobacterias, cianolíquenes, musgosResumen
Las tierras secas constituyen ~41% de la superficie terrestre y sufren degradación, erosión de suelos, disminución de la cobertura y la diversidad de plantas vasculares y de costras biológicas del suelo. Las biocostras son comunidades de organismos como cianobacterias, líquenes, algas y briófitas asociadas a partículas del suelo. Este estudio tiene como objetivo identificar condiciones óptimas de crecimiento de las costras biológicas mediante ensayos de propagación en vivero bajo distintos niveles de luz, humedad y salinidad. En invernadero se cultivaron distintos tipos de costras, dominadas por cianobacterias, cianolíquenes y musgos. Las biocostras fueron cultivadas en macetas bajo tratamientos de radiación (alta, media y baja) y riego (agua destilada cada tres y dos semanas, y riego semanal). A otro grupo de macetas se le agregó sal disuelta en el agua de riego (S1: 0.05 M de NaCl; S2: 0.1 M de NaCl). La cobertura total y por tipo funcional de costras se determinó mediante fotografías de cada maceta utilizando el software libre SamplePoint. Los resultados indicaron que la cobertura total de costras aumentó luego de 24 meses de cultivo y fue superior con los tratamientos de riego semanal para los niveles de radiación media y baja. Las macetas sembradas con musgos alcanzaron mayores coberturas de costras, más del 70%, seguidas por las macetas sembradas con cianobacterias y cianolíquenes. En macetas sin siembra también crecieron costras biológicas, favorecidas por las radiaciones bajas y medias con máximo nivel de riego. Además, al finalizar el ensayo, las cianobacterias crecieron en macetas sembradas con todos los tipos funcionales. El riego con solución salina perjudicó la cobertura de biocostras con respecto a las macetas sin agregado de solución salina. En conclusión, el tipo funcional sembrado, como cianobacterias y musgos, la radiación baja y máxima frecuencia de riego beneficiaron el crecimiento de las costras.
Citas
Adessi, A., R. De Philippis, and F. Rossi. 2021. Drought-tolerant cyanobacteria and mosses as biotechnological tools to attain land degradation neutrality. Web Ecology 21:65-78. https://doi.org/10.5194/we-21-65-2021.
Antoninka, A., A. Faist, E. Rodríguez‐Caballero, K. E. Young, V. B. Chaudhary, L. A. Condon, and D. A. Pyke. 2020a. Biological soil crusts in ecological restoration: emerging research and perspectives. Restoration Ecology 28:S3-S8. https://doi.org/10.1111/rec.13201.
Antoninka, A., M. A. Bowker, N. N. Barger, J. Belnap, A. Giraldo‐Silva, S. C. Reed, F. Garcia-Pichel, and M. C. Duniway. 2020b. Addressing barriers to improve biocrust colonization and establishment in dryland restoration. Restoration Ecology 28:S150-S159. https://doi.org/10.1111/rec.13052.
Antoninka, A., M. A. Bowker, S. C. Reed, and K. Doherty. 2016. Production of greenhouse grown biocrust mosses and associated cyanobacteria to rehabilitate dryland soil function. Restoration Ecology 24:324-335. https://doi.org/10.1111/rec.12311.
Aranibar, J. N., M. J. Repetur, V. R. García, R. Elía Dazat, M. E. Corvalán Videla, and P. E. Villagra. 2022. Functional responses of biological soil crusts to simulated small precipitation pulses in the Monte desert, Argentina. Geoderma 410:115660. https://doi.org/10.1016/j.geoderma.2021.115660.
Barton, K. 2020. MuMIn: Multi-Model Inference. R package version 1.43.17. URL: CRAN.R-project.org/package=MuMIn.
Belnap, J., and D. Eldridge. 2003. Disturbance and recovery of biological soil crusts. Pp. 363-383 en J. Belnap and O. L. Lange (eds.). Biological Soil Crusts: Structure, Function, and Management. Springer, Berlin. https://doi.org/10.1007/978-3-642-56475-8_27.
Belnap, J., B. Büdel, and O. L. Lange. 2001. Biological soil crusts: characteristics and distribution. Pp. 3-30 en J. Belnap and O. L. Lange (eds.). Biological soil crusts: structure, function, and management. Springer, Berlin, Heidelberg.
Belnap, J., S. L. Phillips, and M. E. Miller. 2004. Response of desert biological soil crusts to alterations in precipitation frequency. Oecologia 141:306-316. https://doi.org/10.1007/s00442-003-1438-6.
Booth, D. T., S. E. Cox, and R. D. Berryman. 2006. Point sampling digital imagery with “Samplepoint”. Environmental, Monitoring and Assessment 123:97-108. https://doi.org/10.1007/s10661-005-9164-7.
Bowker, M. A. 2007. Biological soil crust rehabilitation in theory and practice: an underexploited opportunity. Restoration Ecology 15:13-23. https://doi.org/10.1111/j.1526-100X.2006.00185.x.
Bowker, M. A., and A. J. Antoninka. 2016. Rapid ex situ culture of N-fixing soil lichens and biocrusts is enhanced by complementarity. Plant and Soil 408:415-428. https://doi.org/10.1007/s11104-016-2929-7.
Bowker, M. A., A. J. Antoninka, and P. F. Chuckran. 2020. Improving field success of biocrust rehabilitation materials: hardening the organisms or softening the environment? Restoration Ecology 28:S177-S186. https://doi.org/10.1111/rec.12965.
Bowker, M. A., A. J. Antoninka, and R. A. Durham. 2017. Applying community ecological theory to maximize productivity of cultivated biocrusts. Ecological Applications 27:1958-1969. https://doi.org/10.1002/eap.1582.
Bowker, M. A., J. Belnap, V. B. Chaudhary, and N. C. Johnson. 2008. Revisiting classic water erosion models in drylands: the strong impact of biological soil crusts. Soil Biology and Biochemistry 40:2309-2316. https://doi.org/10.1016/j.soilbio.2008.05.008.
Bu, C., R. Li, C. Wang, and M. A. Bowker. 2018. Successful field cultivation of moss biocrusts on disturbed soil surfaces in the short term. Plant and Soil 429:227-240. https://doi.org/10.1007/s11104-017-3453-0.
Canfora, L., E. Vendramin, L. Vittori Antisari, G. Lo Papa, C. Dazzi, A. Benedetti, P. Iavazzo, P. Adamo, A. D. Jungblut, and F. Pinzari. 2016. Compartmentalization of gypsum and halite associated with cyanobacteria in saline soil crusts. FEMS Microbiology Ecology 92(6):fiw080. https://doi.org/10.1093/femsec/fiw080.
Casas, C., M. Brugués, R. M. Cros, and C. Sérgio. 2006. Handbook of Mosses of the Iberian Peninsula and the Balearic Islands. Institut d’Estudis Catalans, Barcelona.
Castenholz, R. W., and F. García-Pichel. 2012. Cyanobacterial responses to UV radiation. Pp. 481-499 en B. Whitton (ed.). Ecology of Cyanobacteria II. Springer, Dordrecht. https://doi.org/10.1007/978-94-007-3855-3_19.
Chamizo, S., A. Adessi, G. Torzillo, and R. De Philippis. 2020. Exopolysaccharide features influence growth success in biocrust-forming cyanobacteria, moving from liquid culture to sand microcosms. Frontiers in Microbiology 11:1-16. https://doi.org/10.3389/fmicb.2020.568224.
Chandler, D. G., N. Day, M. D. Madsen, and J. Belnap. 2019. Amendments fail to hasten biocrust recovery or soil stability at a disturbed dryland sandy site. Restoration Ecology 27:289-297. https://doi.org/10.1111/rec.12870.
Chiquoine, L. P., S. R. Abella, and M. A. Bowker. 2016. Rapidly restoring biological soil crusts and ecosystem functions in a severely disturbed desert ecosystem. Ecological Applications 26:1260-1272. https://doi.org/10.1002/15-0973.
Coe, K. K., J. P. Sparks, and J. Belnap. 2014. Physiological ecology of dryland biocrust mosses. Pp. 291-308 en D. Hanson and S. Rice (eds.). Photosynthesis in bryophytes and early land plants. Springer, Dordrecht. https://doi.org/10.1007/978-94-007-6988-5_16.
Condon, L. A., and D. A. Pyke. 2016. Filling the interspace—restoring arid land mosses: source populations, organic matter, and overwintering govern success. Ecology and Evolution 6:7623-7632. https://doi.org/10.1002/ece3.2448.
Corvalán Videla, M. E., J. N. Aranibar, and S. Greco. 2021. Biological soil crusts from the Monte desert affect soil moisture and nutrients, and improve Leptochloa crinita grass development. Acta Oecologica 110:103712. https://doi.org/10.1016/j.actao.2021.103712.
Delignette-Muller, M. L., and C. Dutang. 2015. fitdistrplus: An R Package for Fitting Distributions. Journal of Statistical Software 64:1-34. https://doi.org/10.18637/jss.v064.i04.
Delmail, D., M. Grube, D. Parrot, J. Cook-Moreau, J. Boustie, P. Labrousse, and S. Tomasi. 2013. Halotolerance in lichens: symbiotic coalition against salt stress. Pp. 115-148 en P. Ahmad, M. Azooz and M. Prasad (eds.). Ecophysiology and responses of plants under salt stress. Springer, New York, NY. https://doi.org/10.1007/978-1-4614-4747-4_4.
Doherty, K. D., A. J. Antoninka, M. A. Bowker, S. V. Ayuso, and N. C Johnson. 2015. A novel approach to cultivate biocrusts for restoration and experimentation. Ecological Restoration 33:13-16. https://doi.org/10.3368/er.33.1.13.
Doherty, K. D., M. A. Bowker, A. J. Antoninka, N. C. Johnson, and T. E. Wood. 2018. Biocrust moss populations differ in growth rates, stress response, and microbial associates. Plant and Soil 429:187-198. https://doi.org/10.1007/s11104-017-3389-4.
Doudle, S., W. Williams, and V. Galea. 2011. Improving rehabilitation outcomes using biocrusts. Pp. 85-97 en Eight International Heavy Minerals Conference 2011, Perth, WA, Australia, 5-6 October 2011. Carlton South, VIC, Australia: Australasian Institute for Mining and Metallurgy (AusIMM).
Eldridge, D. J., and J. F. Leys. 2003. Exploring some relationships between biological soil crusts, soil aggregation and wind erosion. Journal of Arid Environments 53:457-466. https://doi.org/10.1006/jare.2002.1068.
Fattahi, S. M., A. Soroush, and N. Huang. 2020. Wind erosion control using inoculation of aeolian sand with cyanobacteria. Land Degradation and Development 31:2104-2116. https://doi.org/10.1002/ldr.3590.
Flowers, T. J., H. K. Galal, and L. Bromham. 2010. Evolution of halophytes: multiple origins of salt tolerance in land plants. Functional Plant Biology 37:604-612. https://doi.org/10.1071/FP09269.
Gao, L., M. A. Bowker, H. Sun, J. Zhao, and Y. Zhao. 2020. Linkages between biocrust development and water erosion and implications for erosion model implementation. Geoderma 357:113973. https://doi.org/10.1016/j.geoderma.2019.113973.
Garcia, V., J. Aranibar, and N. Pietrasiak. 2015. Multiscale effects on biological soil crusts cover and spatial distribution in the Monte Desert. Acta Oecologica 69:35-45. https://doi.org/10.1016/j.actao.2015.08.005.
Garcia, V., J. Aranibar, and P. E. Villagra. 2021. Propagación de distintos tipos funcionales de la costra biológica del suelo del desierto del Monte, Argentina. Ecología Austral 31:001-016. https://doi.org/10.25260/EA.21.31.1.0.1158.
Glaser, K., K. Baumann, P. Leinweber, T. Mikhailyuk, and U. Karsten. 2018. Algal richness in BSCs in forests under different management intensity with some implications for P cycling. Biogeosciences 15:4181-4192. https://doi.org/10.5194/bg-15-4181-2018.
Green, T. A., and M. C. Proctor. 2016. Physiology of photosynthetic organisms within biological soil crusts: their adaptation, flexibility, and plasticity. Pp. 347-381 en B. Weber, B. Büdel and J. Belnap (eds.). Biological Soil Crusts: An Organizing Principle in Drylands. Ecological Studies, vol 226. Springer, Cham. https://doi.org/10.1007/978-3-319-30214-0_18.
Greco, S. A., C. E. Sartor, and P. E. Villagra. 2013. Minimum water input event for seedling emergence of three native perennial grasses of the Central Monte desert (Argentina) influenced by the effect of shade and the season of the year. Rev Fac Cienc Agrar, Univ Nac Cuyo 45.
Gómez, D. A., J. N. Aranibar, S. Tabeni, P. E. Villagra, I. A. Garibotti, and A. Atencio. 2012. Biological soil crust recovery after long-term grazing exclusion in the Monte Desert (Argentina). Changes in coverage, spatial distribution, and soil nitrogen. Acta Oecologica 38:33-40. https://doi.org/10.1016/j.actao.2011.09.001.
He, X., K. S. He, and J. Hyvönen. 2016. Will bryophytes survive in a warming world? Perspectives in Plant Ecology, Evolution and Systematics 19:49-60. https://doi.org/10.1016/j.ppees.2016.02.005.
Honegger, R. 2012. 15 The symbiotic phenotype of lichen-forming ascomycetes and their endo-and epibionts. Pp. 287-339 en B. Hock (eds.). Fungal Associations. The Mycota, vol. 9. Springer, Berlin, Heidelberg. https://doi.org/10.1007/978-3-642-30826-0_15.
Housman, D. C., H. H. Powers, A. D. Collins, and J. Belnap. 2006. Carbon and nitrogen fixation differ between successional stages of biological soil crusts in the Colorado Plateau and Chihuahuan Desert. Journal of Arid Environments 66:620-634. https://doi.org/10.1016/j.jaridenv.2005.11.014.
Kakeh, J., M. Gorji, M. H. Mohammadi, H. Asadi, F. Khormali, M. Sohrabi, and D. J. Eldridge. 2021. Biocrust islands enhance infiltration, and reduce runoff and sediment yield on a heavily salinized dryland soil. Geoderma 404:115329. https://doi.org/10.1016/j.geoderma.2021.115329.
Komárek, J., and J. R. Johansen. 2015. Filamentous cyanobacteria. Pp. 135-235 en J. D. Wehr, R. G. Sheath and J. P. Kociolek (eds.). Freshwater Algae of North America: Ecology and Classification, Elsevier Academic Press. https://doi.org/10.1016/B978-0-12-385876-4.00004-9.
Lan, S., L. Wu, D. Zhang, and C. Hu. 2012. Successional stages of biological soil crusts and their microstructure variability in Shapotou region (China). Environmental Earth Sciences 65:77-88. https://doi.org/10.1007/s12665-011-1066-0.
Liu, Y. R., M. Delgado-Baquerizo, P. Trivedi, J. Z. He., J. T. Wang, and B. K. Singh. 2017. Identity of biocrust species and microbial communities drive the response of soil multifunctionality to simulated global change. Soil Biology and Biochemistry 107:208-217. https://doi.org/10.1016/j.soilbio.2016.12.003.
Maestre, F. T., M. A. Bowker, Y. Canton, A. P. Castillo-Monroy, J. Cortina, C. Escolar, A. Escudero, R. Lazaro, and I. Martínez. 2011. Ecology and functional roles of biological soil crusts in semi-arid ecosystems of Spain. Journal of Arid Environments 75:1282-1291. https://doi.org/10.1016/j.jaridenv.2010.12.008.
Nash III, T. H., B. D. Ryan, P. Diederich, C. Gries, and F. Bungartz. 2002. Lichen Flora of the Greater Sonora Desert Region, vol 2. Lichen Unlimited, Tempe, Arizona, USA.
Rastogi, R. P., R. R. Sonani, and D. Madamwar. 2014. The high-energy radiation protectant extracellular sheath pigment scytonemin and its reduced counterpart in the cyanobacterium Scytonema sp. R77DM. Bioresource Technology 171:396-400. https://doi.org/10.1016/j.biortech.2014.08.106.
Reynolds, J. F., D. M. S. Smith, E. F. Lambin, B. L. Turner, M. Mortimore, S. P. Batterbury, T. E. Downing, H. Dowlatabadi, R. J. Fernández, J. E. Herrick, E. Huber-Sannwald, H. Jiang, R. Leemans, T. Lynam, F. T. Maestre, M. Ayarza, and B. Walker. 2007. Global desertification: building a science for dryland development. Science 316:847-851. https://doi.org/10.1126/science.1131634.
Rodríguez-Caballero, E., J. Belnap, B. Büdel, P. J. Crutzen, M. O. Andreae, U. Pöschl, and B. Weber. 2018. Dryland photoautotrophic soil surface communities endangered by global change. Nature Geoscience 11:185-189. https://doi.org/10.1038/s41561-018-0072-1.
Roncero-Ramos, B., J. R. Román, C. Gómez-Serrano, Y. Cantón, and F. G. Acién. 2019. Production of a biocrust-cyanobacteria strain (Nostoc commune) for large-scale restoration of dryland soils. Journal of Applied Phycology 31:2217-2230.
Rosentreter, R. 2020. Biocrust lichen and moss species most suitable for restoration projects. Restoration Ecology 28:S67-S74. https://doi.org/10.1111/rec.13082.
Rossi, F., R. M. Potrafka, F. G. Pichel, and R. De Philippis. 2012. The role of the exopolysaccharides in enhancing hydraulic conductivity of biological soil crusts. Soil Biology and Biochemistry 46:33-40. https://doi.org/10.1016/j.soilbio.2011.10.016.
R Core Team. 2021. R: A language and environment for statistical computing. R Foundation for Statistical Computing, Vienna, Austria. URL: R-project.org.
Sand-Jensen, K., and T. S. Jespersen. 2012. Tolerance of the widespread cyanobacterium Nostoc commune to extreme temperature variations (-269 to 105 °C), pH and salt stress. Oecologia 169:331-339. https://doi.org/10.1007/s00442-011-2200-0.
Singh, H. 2018. Desiccation and radiation stress tolerance in cyanobacteria. Journal of Basic Microbiology 58:813-826. https://doi.org/10.1007/s00442-011-2200-0.
Singh, R. P., P. Yadav, R. Kujur, K. D. Pandey, and R. K. Gupta. 2022. Cyanobacteria and salinity stress tolerance. Pp. 253-280 en P. Singh, M. Fillat and A. Kumar (eds.). Cyanobacterial Lifestyle and Its Applications in Biotechnology. Academic Press. https://doi.org/10.1016/B978-0-323-90634-0.00003-2.
Slate, M. L., R. A. Durham, and D. E. Pearson. 2020. Strategies for restoring the structure and function of lichen‐moss biocrust communities. Restoration Ecology 28:S160-S167. https://doi.org/10.1111/rec.12996.
Steffen, W., K. Richardson, J. Rockström, S. E. Cornell, I. Fetzer, E. M. Bennett, R. Biggs, S. R. Carpenter, W. de Vries, C. A. de Wit, C. Folke, D. Gerten, J. Heinke, G. M. Mace, L. M. Persson, V. Ramanathan, B. Reyers, and S. Sörlin. 2015. Planetary boundaries: Guiding human development on a changing planet. Science 347(6223):1259855. https://doi.org/10.1126/science.1259855.
Tamaru, Y., Y. Takani, T. Yoshida, and T. Sakamoto. 2005. Crucial role of extracellular polysaccharides in desiccation and freezing tolerance in the terrestrial cyanobacterium Nostoc commune. Applied and Environmental Microbiology 71:7327-7333. https://doi.org/10.1128/AEM.71.11.7327-7333.2005.
Velasco Ayuso, S., A. Giraldo Silva, C. Nelson, N. N. Barger, and F. García-Pichel. 2017. Microbial nursery production of high quality biological soil crust biomass for restoration of degraded dryland soils. Applied and Environmental Microbiology 83:e02179-16. https://doi.org/10.1128/AEM.02179-16.
Velasco Ayuso, S. V., A. Giraldo‐Silva, N. N. Barger, and F. García‐Pichel. 2020. Microbial inoculum production for biocrust restoration: testing the effects of a common substrate versus native soils on yield and community composition. Restoration Ecology 28:S194-S202. https://doi.org/10.1111/rec.13127.
Weber, B., B. Büdel, and J. Belnap. 2016. Biological soil crusts: an organizing principle in drylands. Springer, Berlin Heidelberg New York. https://doi.org/10.1007/978-3-319-30214-0.
Wong, C. S., and W. K. Li. 1998. A note on the corrected Akaike information criterion for threshold autoregressive models. Journal of Time Series Analysis 19:113-124. https://doi.org/10.1111/1467-9892.00080.
Xiao, B., Y. Zhao, Q. Wang, and C. Li. 2015. Development of artificial moss-dominated biological soil crusts and their effects on runoff and soil water content in a semi-arid environment. Journal of Arid Environments 117:75-83. https://doi.org/10.1016/j.jaridenv.2015.02.017.
Zabala, C., J. Aranibar, and D. Rodríguez. 2023. Las comunidades criptógamas como potenciales indicadores de recuperación post-fuego en el pedemonte. Ecología Austral 33(1):108-123. https://doi.org/10.25260/EA.23.33.1.0.1820.
Zhang, W., S. Liu, C. Li, P. Zhang, and P. Zhang. 2019. Transcriptome sequencing of Antarctic moss under salt stress emphasizes the important roles of the ROS-scavenging system. Gene 696:122-134. https://doi.org/10.1016/j.gene.2019.02.037.
Zhao, Y., and M. Xu. 2013. Runoff and soil loss from revegetated grasslands in the hilly Loess Plateau region, China: influence of biocrust patches and plant canopies. Journal of Hydrologic Engineering 18:387-393. https://doi.org/10.1061/(ASCE)HE.1943-5584.0000633.
Zhou, X., Y. Zhao, J. Belnap, B. Zhang, C. Bu, and Y. Zhang. 2020. Practices of biological soil crust rehabilitation in China: experiences and challenges. Restoration Ecology 28:S45-S55. https://doi.org/10.1111/rec.13148.
Zhou, X., T. Ke, S. Li, S. Deng, X. An, X. Ma, R. De Philippis, and L. Chen. 2020. Induced biological soil crusts and soil properties varied between slope aspect, slope gradient and plant canopy in the Hobq desert of China. Catena 190:104559. https://doi.org/10.1016/j.catena.2020.104559.
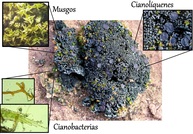
Descargas
Archivos adicionales
Publicado
Cómo citar
Número
Sección
Licencia
Derechos de autor 2023 Vanesa R. Garcia, Julieta N. Aranibar, Pablo E. Villagra

Esta obra está bajo una licencia internacional Creative Commons Atribución 4.0.
Las/os autoras/es conservan sus derechos de autoras/es: 1) cediendo a la revista el derecho a su primera publicación, y 2) registrando el artículo publicado con una Licencia de Atribución de Creative Commons (CC-BY 4.0), lo que permite a autoras/es y terceros verlo y utilizarlo siempre que mencionen claramente su origen (cita o referencia incluyendo autoría y primera publicación en esta revista). Las/os autores/as pueden hacer otros acuerdos de distribución no exclusiva siempre que indiquen con claridad su origen, así como compartir y divulgar ampliamente la versión publicada de su trabajo.